Chapter 4 Measuring Geological Time
Principles of Relative Dating
The process of determining the relative order of past geologic events without necessarily determining their absolute (numeral) age is known as relative dating. This method was developed in the 18th century when geology first emerged as a natural science. To determine the sequence of geologic events, several principles must be followed. Though these principles will seem common sense once you learn them, they were ground breaking in many ways, and are still used today in the field of geology.
Understanding the Difference Between Relative and Absolute Ages
It is important to understand the fundamental differences between relative and absolute ages. Relative ages are simply the sequence of events in the order they occurred, but provide no information about what time each event occurred or the amount of time between events. Absolute ages are numerical ages. They tell us when an event occurred, usually determined from the decay or radioactive isotopes. It is important to know that geoscientists developed relative age dating techniques and were able to put together the geologic history of Earth long before discovery of radioactive decay made determination of numerical ages even possible. However, without numerical ages attached to the geologic sequence of events, it was impossible to how long ago these events occurred or what the rates of geologic processes even were. We only simply knew that geologic processes take a long time. However, by combining relative and absolute geologic ages, we are now able to determine important information such as the tempo of supereruptions of the Yellowstone Caldera System, or the time between major earthquakes on faults near urban areas.
As an example of the difference between relative and absolute ages, consider my routine on a nice winter weekday:
Relative sequence of events | Absolute age of events |
1. Woke up | 5:00 AM |
2. Fed my dogs | 5:30 AM |
3. Went for a quick nordic ski before work | 6:00 AM |
4. Took a shower and got dressed | 8:00 AM |
5. Drove to work | 8:40 AM |
6. Taught a geology lecture | 9:00 AM |
7. Ate lunch | 11:00 AM |
8. Read some papers about geologic faults | 12:00 PM |
9. Drove home | 4:30 PM |
10. Made dinner | 7:00 PM |
11. Read a book | 8:00 PM |
12. Brushed my teeth and went to bed | 10:00 PM |
From this example, you can see that a lot of information can still be gathered about my day just from the relative sequence of events. However, without the element of time attached, it is impossible to know the rate at which I completed tasks. You can also see some events cannot occur out of order (e.g., I can’t teach my geology lecture before I wake up and get out of bed).
Uniformitarianism
The principle of uniformitarianism was first stated by James Hutton in the 18th century, and is a fundamental underpinning of our understanding of geologic processes. Hutton realized the events in the earths past can be understood by observing the processes we see happening today. Natural processes such as erosion and deposition, plate tectonics, and the laws of gravity and isostasy produce the features of the Earth. These processes have not changed. For example, the processes that transport and deposit sediment in a river are the same today as they were a billion years ago (Figure 4.2.1). Therefore the principle of uniformitarianism is expressed as “the present is the key to the past” which guides the principles of stratigraphy.
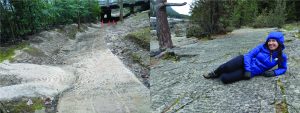
The Principle of Superposition
In a series of undisturbed layered rocks, each successive layer above is younger than the layer below it. Therefore, the oldest rocks are at the bottom of the sequence and the youngest are at the top. This is pretty straight forward in practice. In Figure 4.2.2 (left) you can a cliff with horizontal layers of sandstone. The light colored layer at the base of the cliff was deposited first because it is at the bottom, and each layer above was deposited in order from bottom to top. Making the uppermost layer the youngest.
The Principle of Original Horizontality
Sediments deposited or precipitated on the Earth’s surface are under the influence of gravity, and will accumulate in horizontal layers (Figure 4.2.2 left). This is also generally true of most lava flows or volcanic ash that is erupted and deposited at Earth’s the surface. Therefore, if rocks are tilted, folded or metamorphosed, then these events must have followed deposition and lithification. For example, in Figure 4.2.2 (right), rocks that were originally deposited in horizontal layers like the cliffs in Figure 4.2.2 (right). They were then subsequently subjected to heat and pressure, resulting in transformation to contorted, tilted, and folded marble.
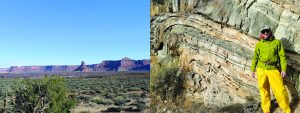
The Principle of Lateral Continuity
Sediments are deposited laterally in continuous layers. Therefore if horizontal strata are dissected by erosional features, such as a valley, they can be interpreted to be the same strata (Figure. 4.2.3 left). Imagine you are at Dead Horse Point near Moab, Utah (Figure 4.2.3 right) or even the rim of the Grand Canyon. The horizontal layers of rock that are dissected by the Colorado River can be interpreted to be the same stratigraphic layers on the other side of canyon. Furthermore, you can begin to put together the relative age of geologic events of such a view, even from the few principles we have learned: 1) Each layer was deposited in order from bottom to top, so the oldest layers are at the bottom, youngest at the top, 2) the layers are still more or less horizontal so, so no major geologic event has tilted or contorted these rocks, 3) subsequent to depositing all of the layers you can see at this view point, the Colorado River cut down through the layers. You can see this starts to become simple logic. The Colorado River couldn’t cut down through layers if they didn’t exist. Therefore, all the layers that Colorado River cuts down through had to have been deposited before the Colorado River began cutting down through them and forming this canyon.

The Principle of Cross-Cutting Relationships
Any geologic feature that crosscuts or modifies another feature must be younger than the rocks it cuts through. The cross-cutting feature is the younger feature because there must be something previously there to cross-cut. Cross-cutting features can include folds, faults, and igneous intrusions. They can also include events like metamorphism (Figure 4.3.4).
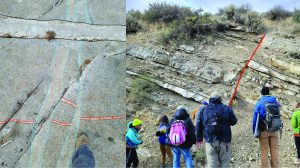
The Principle of Baked Contacts
This principle is similar to the Principle of Cross-Cutting Relationships. Magma will heat/metamorphose or “bake” the rocks it comes in contact with. Therefore if the rocks surrounding an igneous rock have a “baked contact”, they must have been present before the magma cooled (Figure 4.3.5).
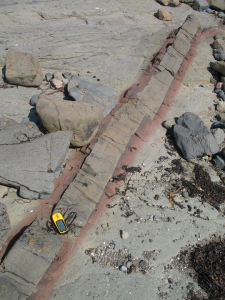
The Principle of Inclusions
The principle of inclusions states that any rock fragments that are included in rock must be older than the rock in which they are included. For example, a xenolith in an igneous rock or a clast in sedimentary rock must be older than the rock that includes it (Figure 4.3.6).
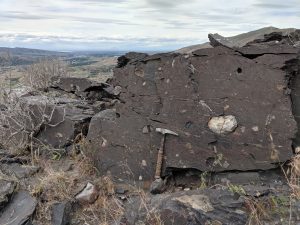
Exercise 4.1 Cross-Cutting Relationships
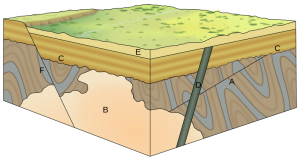
Can you put all of the principles of relative age dating to work and solve the geologic puzzle in Figure 4.3.7? Describe in order the sequence of events that occurred in the diagram, listing them in order from oldest to youngest.
See Appendix 3 for Exercise 4.1 answers.
Unconformities
An unconformity represents an interruption in the process of deposition of sedimentary rocks. Recognizing unconformities is important for understanding time relationships in sedimentary sequences. An example of an unconformity is shown in Figure 4.3.8. The Proterozoic rocks of the Grand Canyon Group have been tilted and then eroded to a flat surface prior to deposition of the younger Paleozoic rocks. The difference in time between the youngest of the Proterozoic rocks and the oldest of the Paleozoic rocks is close to 300 million years. Tilting and erosion of the older rocks took place during this time, and if there was any deposition going on in this area, the evidence of it is now gone.
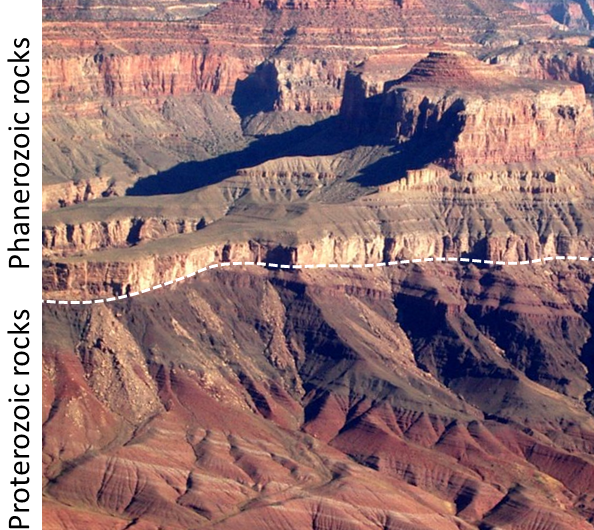
There are four types of unconformities, as summarized in Table 4.1, and illustrated in Figure 4.3.9.
Unconformity Type | Description |
---|---|
Nonconformity | A boundary between non-sedimentary rocks (below) and sedimentary rocks (above) |
Angular unconformity | A boundary between two sequences of sedimentary rocks where the underlying ones have been tilted (or folded) and eroded prior to the deposition of the younger ones (as in Figure 4.3.8) |
Disconformity | A boundary between two sequences of sedimentary rocks where the underlying ones have been eroded (but not tilted) prior to the deposition of the younger ones (as in Figure 4.3.9 c) |
Paraconformity | A time gap in a sequence of sedimentary rocks that does not show up as an angular unconformity or a disconformity |
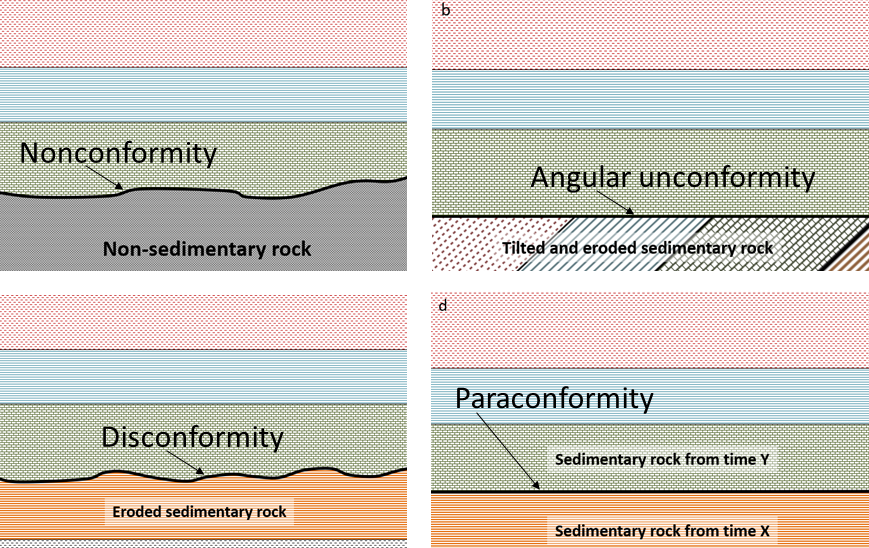
Media Attributions
- Physical Geology Laboratory by Cindy Kearns and Elizabeth Johnson is licensed under CC BY SA 4.0, adapted from the original by Ryan B. Anderson.
- Physical Geology-2nd Edition, by Steven Earle is licensed under CC BY 4.0, Modified from the original by Ryan B. Anderson.
- Figures 4.3.1, 4.3.2, 4.3.3 (right), 4.3.4, 4.3.6: © Steven Earle. CC BY.
- Figure 4.3.3 (left) Woudloper. Public Domain.
- Figure 4.3.5. © John Faithfull. CC BY.
- Figure 4.3.7: Woudloper. CC BY SA.
- Figures 4.3.8, 4.3.9: © Steven Earle. CC BY.
a boundary in rock or sediment along which displacement has taken place
the principle that inclusions within a body of rock must be older than the rock
a fragment of country incorporated into igneous rock, commonly as a result of stoping
a geological boundary at the base of a sedimentary layer